The genetic secrets of survivor bees
Research at the Center for Integrative Bee Research (CIBER) at the University of California Riverside focuses on unique genes in the honey bee genome of Southern California’s survivor bees. These bees have adapted to thrive in challenging environments without human intervention, demonstrating resilience to diseases, climate extremes, and urbanised or intensively farmed landscapes. In this second article in our series, geneticist Christopher Allen uncovers the mechanisms behind their resilience. These discoveries will not only help identify bee genes responsible for these survival traits, but also pave the way for breeding stress-resilient bees which can provide essential pollination services in the future.
Decoding the bee genome
To unravel the secrets of survivor bees, we first need to understand their origins and genetic makeup. Inspired by consumer-based genome analysis companies like Ancestry and Embark, where many people easily provide saliva samples to glimpse their genetic information, researchers at CIBER are applying similar approaches to honey bees.
Historical records have documented the importation of several honey bee lineages from the ancestral range (Europe, the Middle East and Africa) into the USA. We now know that these ancestral lineages have been interbreeding and mixing up genetics across the country, creating populations that are distinct from their ancestors. Southern California’s survivor bees are a product of this mixing, and their genomes reflect the evolutionary forces of natural selection driving their fitness in challenging environments.
What is genetic diversity and why does it matter?
Genetic diversity refers to variations in DNA sequences within a population, providing a ‘toolkit’ for adaptation to changing environments. For example, the human genome, which is made up of three billion base pairs, is tightly coiled within each cell. If stretched out, its length would measure six feet for every individual, regardless of their height. Remarkably, 99.9% of our genetic code is identical from one person to the next, with the vast differences we observe arising from just 0.1% of variation.
For honey bees, with genomes comprising 260 million base pairs, even small genetic changes can significantly impact observable traits. Researchers measure genetic diversity using tools like ancestry analysis and nucleotide diversity. Ancestry analysis maps the historical flow of genes across populations, revealing connections and divergences over time. Nucleotide diversity, on the other hand, is a more granular measure, homing in on variations at specific DNA sites and highlighting differences that drive adaptation and evolution. By combining these approaches, we can trace a population’s history, understand its present-day resilience, and predict its responses to future challenges.
Tools of the trade: sequencing technologies
Now, how do we measure something so intangibly small and complex as DNA sequences? If you want to measure height, you could grab a tape measure and start recording. But DNA sequences aren’t visible, and they’re made up of millions to billions of tiny ‘letters’ (A, T, C and G) that are referred to as the genetic code. To read DNA, we rely on sequencing machines, which can capture these letters in a variety of ingenious ways at mind boggling fast speeds.
For example, Oxford Nanopore’s sequencers measure tiny changes in electrical currents as each individual DNA nucleotide that passes through a small hole in the machine. It’s like reading a squiggle of electrical signals that can be converted into the DNA sequence and it’s reading those squiggles at a dizzying rate of 400 bases per second.
Another sequencing technology we use relies on flashes of colour. Here, fluorescent tags are attached to each DNA base, and, as the machine reads the sequence, each base gives off a specific colour that the machine then reads as an individual DNA nucleotide. Imagine watching fireworks where each colour represents a letter in the genetic code. These approaches allow scientists to ‘read’ the invisible, revealing DNA’s secrets one base at a time, and driving discoveries in fields from medicine to agriculture.
Unlike traditional genetic studies that analyse small fragments or focus on one or a few genes, whole genome sequencing (using platforms such as ONT or Illumina) enables us to decode the entire honey bee genome. This approach provides a much more comprehensive view of the genetic information, allowing us to explore the full scope of genetic diversity. By examining complete genomes, we can uncover critical insights into the genetic basis of traits that make survivor bees unique and better understand how these traits are distributed across populations.
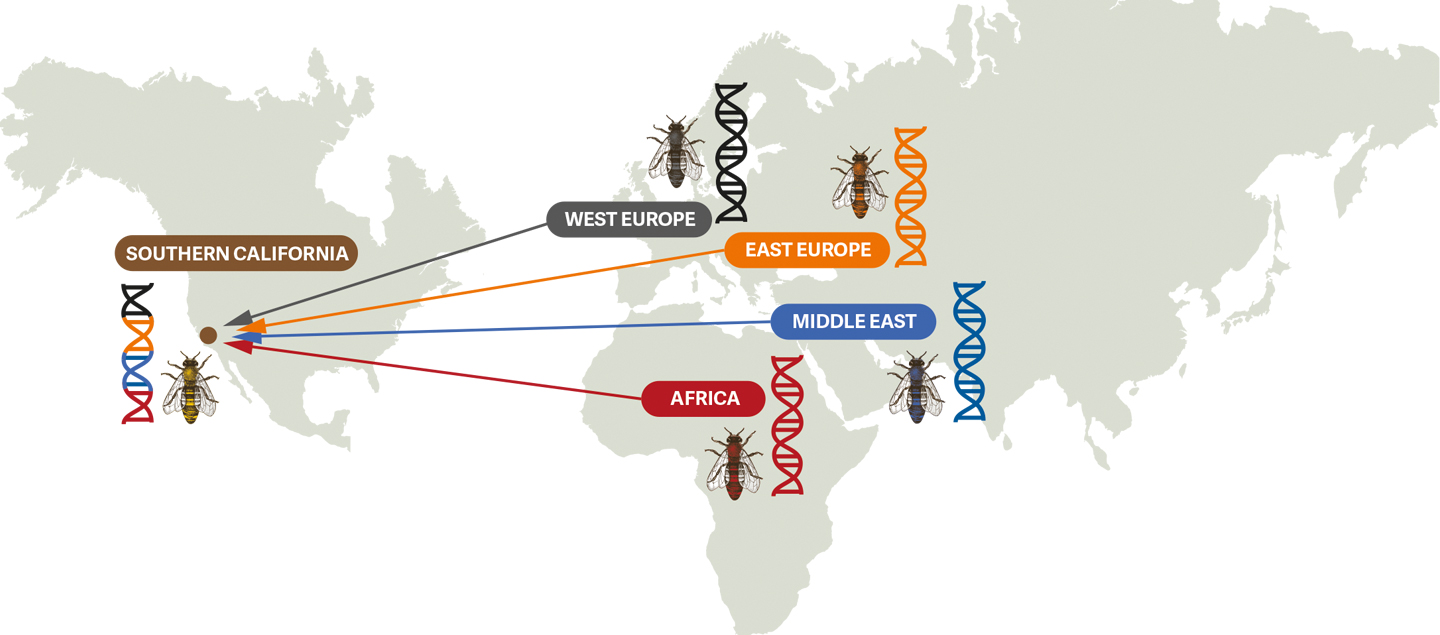
Genetic mixing among survivor bees. Ancestry analysis has detected four major ancestral groups which are present in the survivor bee genome.
Utilising reference genomes
How do geneticists make meaningful comparisons from DNA samples? It all starts with a reference genome – a detailed map of an organism’s genetic blueprint. For honey bees (Apis mellifera), the first reference genome was published in 2006. Thanks to advances in sequencing technology, this was updated in 2019, providing a more comprehensive ’gold standard’ that serves as the foundation for assembling and analysing individual genomes. Every sample we analyse is constructed with this reference genome as a guide, ensuring consistency and comparability across datasets. This essential step allows scientists to accurately identify genetic variations and draw meaningful conclusions about evolutionary history, genetic diversity and ancestry.
A wealth of genetic data is available through the National Center for Biotechnology Information (NCBI), an online repository where scientists share and access billions of genetic records. For our research, we harness this resource to study Apis mellifera samples collected globally, using them to build an ancestral panel. It’s important to note that the ancestral panel is not a reference genome, but rather a collection of deeply sequenced genomes from individuals across Europe, Africa and the Middle East at 60-times coverage for high accuracy. This means that the machine that sequenced the honey bees’ DNA repeated the tests 60 times to increase accuracy, because even these highly sophisticated systems can make errors. These sequences, aligned to the reference genome, allow us to model ancestral genetic variation and trace the genetic makeup of Southern California’s survivor bees.
What can the genetic secrets of survivor bees tell us?
Understanding genetic diversity in bees isn’t just a scientific exercise; it holds real implications for the future of beekeeping and agriculture. By uncovering how different populations of bees have adapted over generations, we gain insights into their resilience against challenges like climate change, disease and habitat loss.
With the creation of a population-specific reference genome for Southern California, we could better understand how the environment is shaping genomes across microclimates. If we determine, for example, that coastal areas, mountain ranges and deserts all have locally adapted stock, this knowledge can guide breeding programmes to select for hardy, adaptable bees that thrive in a variety of environments. This could have wider repercussions for commercial beekeepers who may wish to provide premium pollination services or breed a specialised commercial stock for certain regions.
Ultimately, by decoding the invisible layers of bee diversity, we’re laying the groundwork for healthier hives and more sustainable beekeeping practices that can support food security and biodiversity worldwide.
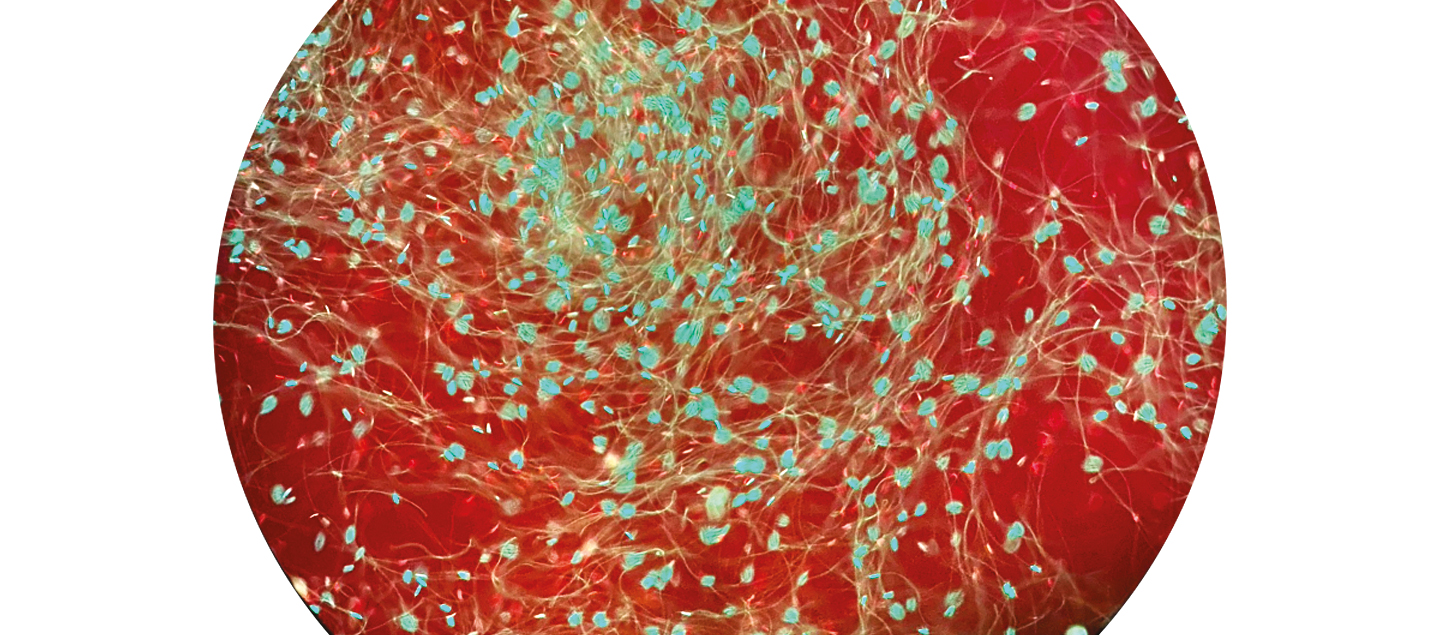
How do survivor bees cope with the heat?
For my research into honey bee thermotolerance, or how well bees handle heat-related stress, I am measuring and analysing heat shock proteins (HSPs) both before and after exposure to very high temperatures. These HSPs are found in the seminal fluid of drones so their thermotolerance can be measured by counting the number of viable sperm. In this sample, from an imported commercial colony, live cells are stained green and dead sperm appear red (see below).
My preliminary findings suggest that there is a diversity in heat shock variance within honey bee colonies: the commercial colonies (European imports) experience a drop in fertility after being exposed to heat that we do not observe in the Southern Californian group. What’s more, I detected a variant that was not present in any recorded ancestral data in a colony I collected from Palm Springs, a city in the Sonoran Desert of Southern California where temperatures can reach 49C in summer.
By comparing new variants like these to those in the DNA of commercial stock and their ancestors (the chosen reference genome), I hope to determine what causes this thermotolerance in the Southern Californian population.
In my role as a genetic detective, I seek answers to the question: did these survivor bees adapt to the local climate or are these indicators of thermotolerance somehow missing from the comprehensive ancestral data?
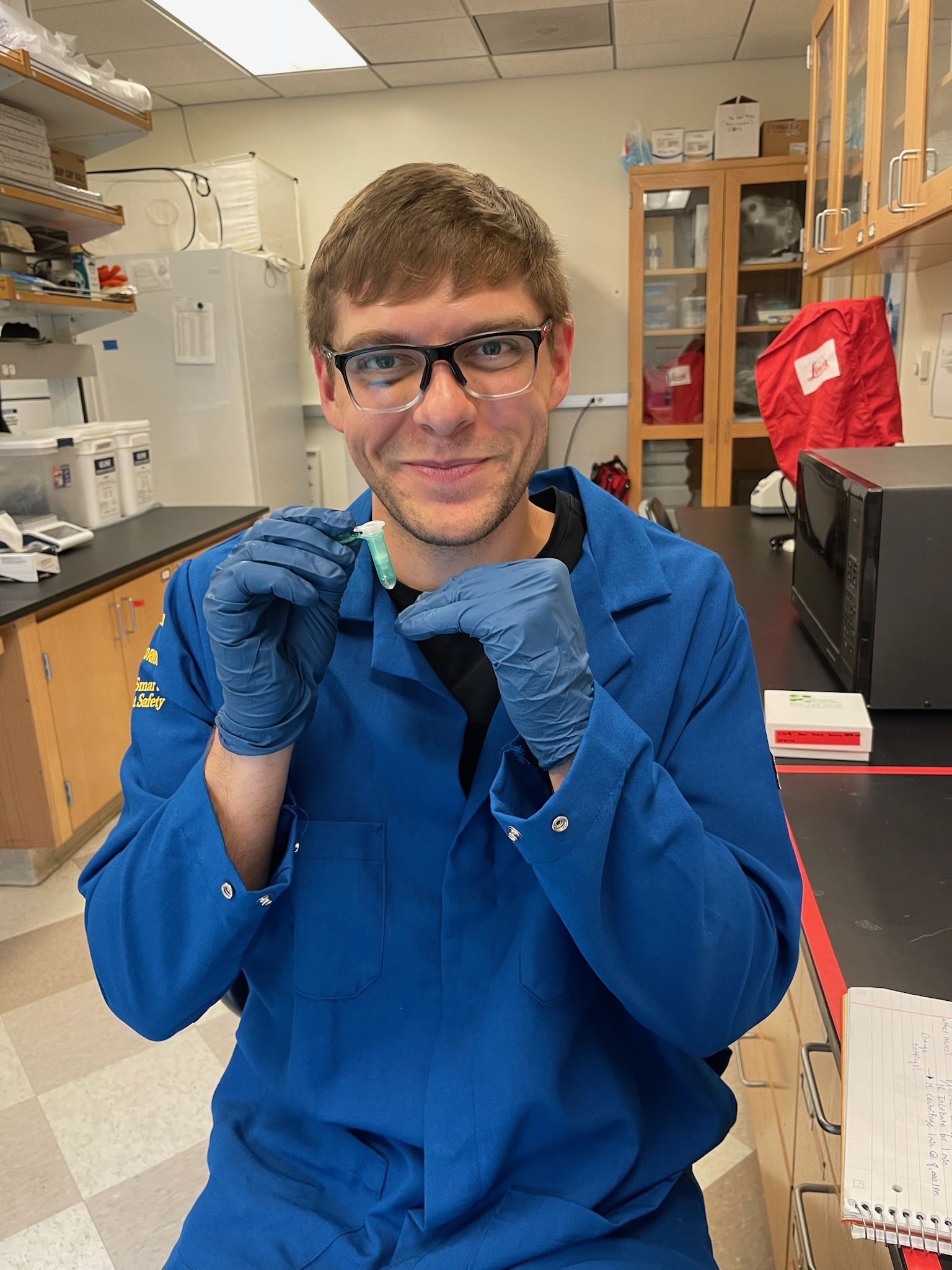
Christopher Allen
Christopher Allen is a PhD candidate at the University of California, Riverside (UCR), affiliated with the Center for Integrative Bee Research (CIBER) and supervised by Prof Boris Baer. His research focuses on the comparative proteomics of honey bees from distinct genetic lineages, aiming to understand how genetic diversity and hybridisation among Southern California honey bees contribute to resilience against heat stress.